I am interested in understanding planets and the architecture in which they form and evolve. In practice, this means observing and modeling populations of exoplanets and trying to piece together their dynamical histories. I am particularly interested in understanding the origin of hot Jupiters, or massive planets on very short-period orbits. These planets were some of the very first discovered around other stars and have no analog in our solar system. I’ve described a few of the projects I work on below, but you can find a list of all my published work here.
Header image credit: ESA/ATG Medialab
Companions to Warm Jupiters
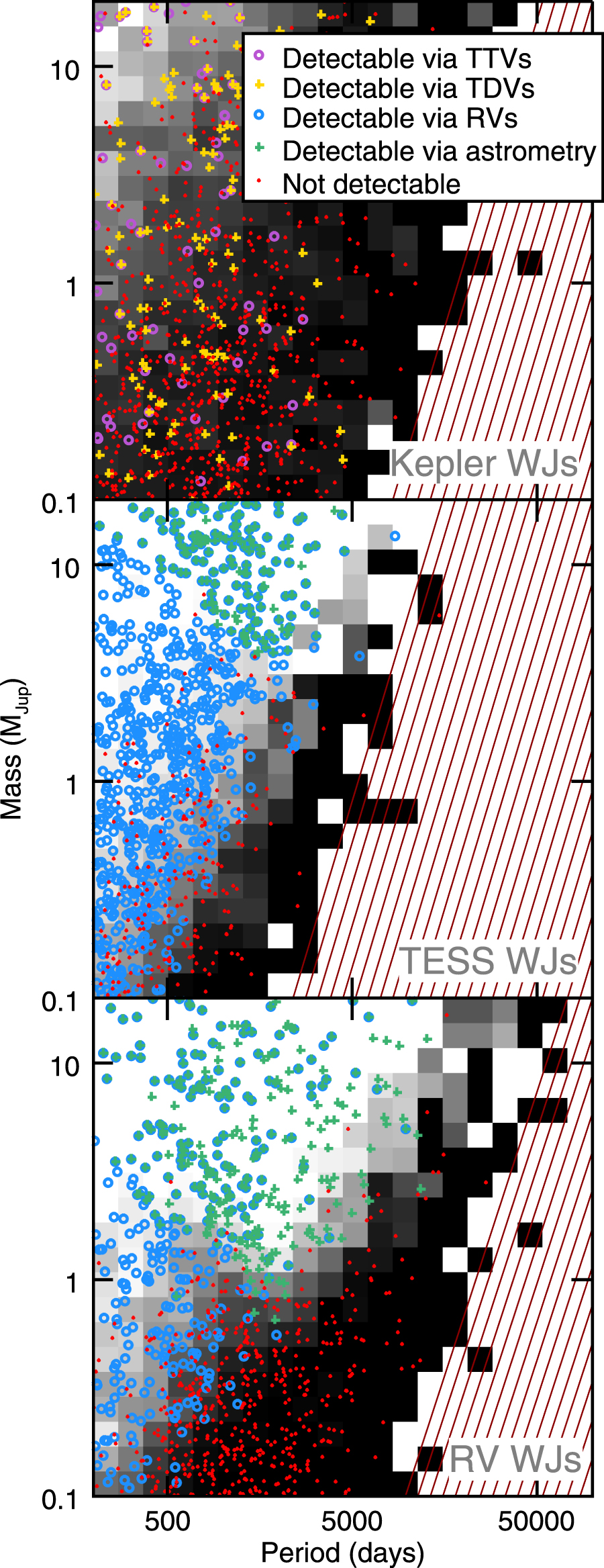
We think it is unlikely that (at least most) hot Jupiters formed on the orbits we currently observe. Instead, they likely formed further out in their stellar systems and migrated inwards. This idea sets up warm Jupiters (i.e., massive planets on slightly less extreme orbits) as potential intermediaries caught in the act of migrating. If warm Jupiters are in fact “proto-hot Jupiters”, we can learn a lot about the migration process by studying them. However, the difficulty lies in the fact that we are less certain warm Jupiters require migration to reach their current orbits – they may instead be an entirely independent population of planets from the hot Jupiters.
If warm Jupiters are actively migrating, they require massive, nearby companion planets or stars to exchange angular momentum with. In exoplanet science, “massive” and “nearby” often translate to “detectable” since the main methods of planet detection favor large, short-period planets. Given some assumptions about what the population of companions might look like, we can predict how likely we are to detect these companions if they exist. In Jackson et al. (2021), we showed that these companions should largely be detectable in warm Jupiter systems discovered by the TESS telescope.
We are now starting to test this prediction with new data! If we do not find a significant population of massive, nearby planets in warm Jupiter systems, it may imply that most warm Jupiters did not migrate to their current orbits and are therefore not proto-hot Jupiters. If, instead, we do find evidence of this population, we can follow up on individual systems to determine how likely they each are to have engaged in migration.
TESS Single-transit Planets
The Transiting Exoplanet Survey Satellite (TESS) is an excellent tool for discovering short-period planets across the entire sky. However, most stars are only observed for about 27 days at a time. Since we typically require 3 transit signals to detect a planet, longer-period orbits may be missed. That said, with even a single observed transit, we can learn some things about the potential planet that caused the signal.
Here at Van Vleck Observatory, we are using our automated, student-run 24″ telescope to monitor TESS targets with single-transit events. If we detect additional transits, we can begin to narrow down the period of the planet and target it for follow-up observations. As an example of what this might look like, here is a paper I contributed to announcing the discovery of a planet first detected as a single-transit signal by TESS.
Where are all the super-eccentric Jupiters?
There are two primary theories for how hot Jupiters may have migrated to their current orbits. The first is smooth migration through the disk of gas surrounding the star early in its lifetime. The second is called “high-eccentricity migration” and involves the planet being excited to an extremely elliptical orbit and subsequently losing orbital energy through tides raised in the planet by the star.
In this second scenario, we should see a steady-state of extremely elliptical planets in the process of migrating to become hot Jupiters. In Jackson et al. (2023), we showed that we don’t see enough planets on this type of extreme orbit to account for the majority of the hot Jupiter population. Thus, another migration mechanism is necessary to explain the observations.
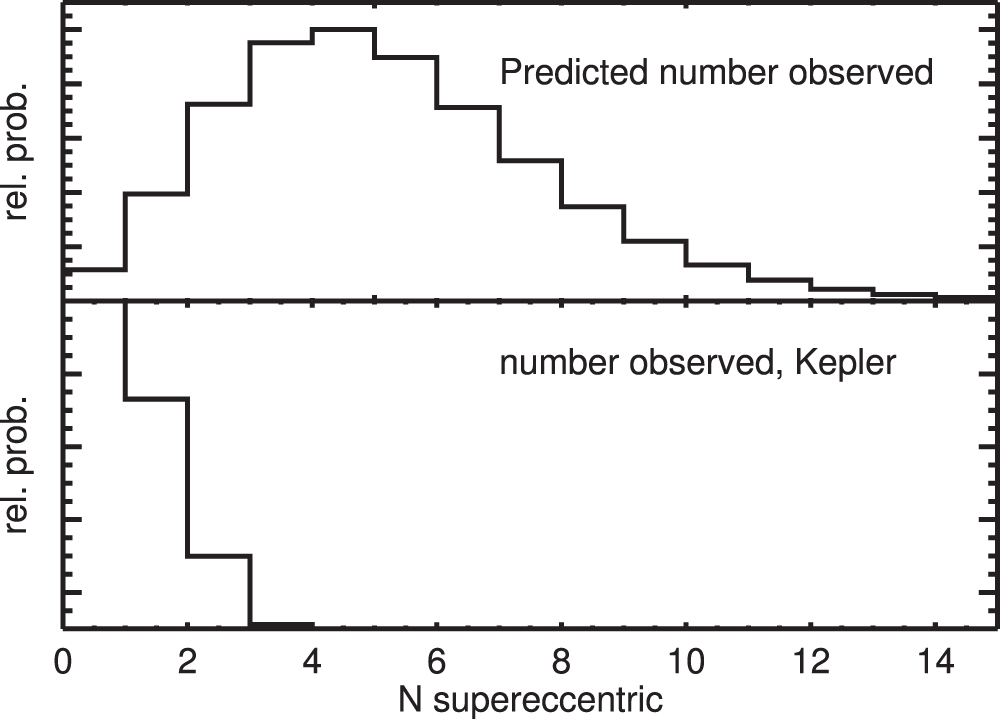